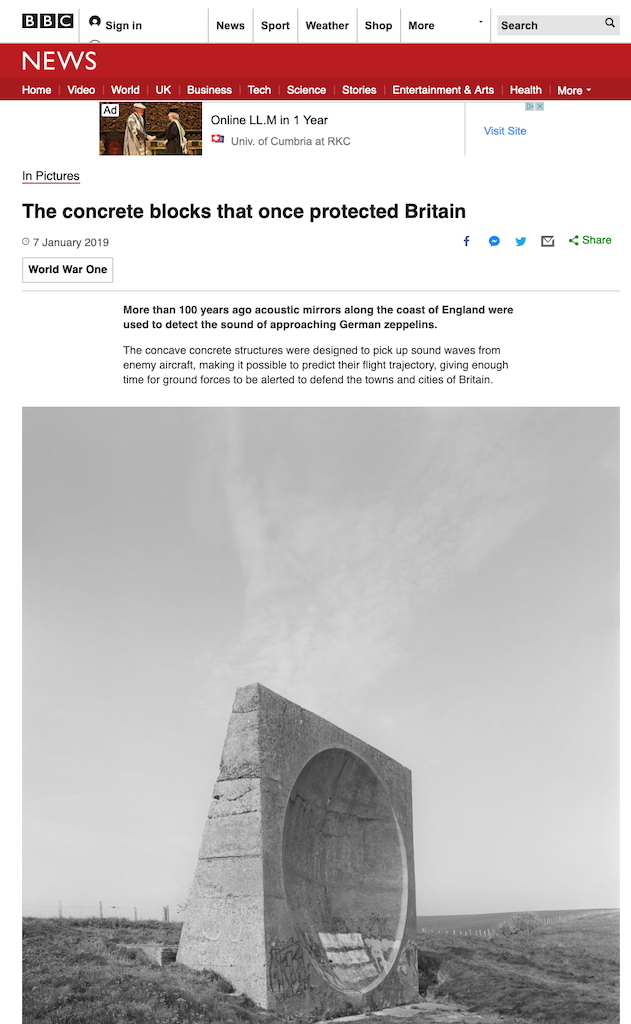
Category: audio
“Still I ought to please any passing bat with my high fidelity”
“…surprised they let you have it in this room anyway… the aCOWstics are all wrong… If you raise the ceiling 4 feet, put the far place on that wall to that wall, you’ll still only get the stereophonic effect if you sit in the bottom of that cupboard…”
Only in Canada?
Xmas list
Martian acoustics (sort of…)
Parisien acoustics
How your ear works
Siegfried Linkwitz (1935-2018)
I love two of the quotes in this nice obituary at Stereophile:
‘… some things that look gross in the frequency response, the ear says, “I don’t care”.’
– Siegfried Linkwitz
“Measurements are useful tools, but don’t let them hold you hostage.”
– Michael Fremer
Jitter – Part 9 – When do I care?
#9 in a series of articles about wander and jitter
In order to talk about WHEN we care about jitter, we have to separate jitter into the categories of Data Jitter and Sampling Jitter
Data Jitter
In the case of Data Jitter, our only real worry is that the data transmission doesn’t get bit errors. In almost all cases, this should be taken care of by the equipment itself – or the components inside it. If you have a device with a digital output, hopefully, that output has been tested to ensure that it meets the standards set for it. If it’s an AES/EBU output, then it meets those standards. If it’s an S-PDIF coaxial output, then it meets those standards. This doesn’t just mean that the data coming out of that output is correct. It also means that the output impedance of the hardware is correct, the voltage levels are correct, and so on. They have to meet the standard requirements. This is easily testable if you have the correct equipment. I won’t mention any brands here because there are many.
The same is true for a digital input. Either it meets the appropriate standard, and it works, or it doesn’t – and this will be the fault of the manufacturer and the supplier of the components inside. However, again, the input must have the correct input impedance, be able to accept the correct voltage ranges, and meet the specifications for the transmission protocol with respect to jitter immunity. This is one of the nice things about digital audio transmission protocols like AES/EBU and S-PDIF. The standards assume that there will be some jitter in the transmission system, and the receiver must be able to withstand this (remember we’re specifically talking about data jitter here). This is tested by intentionally adding jitter to a signal sent to the device, and looking at the errors at its output. The standards state thresholds for jitter – meaning that if you do induce (or accidentally have) jitter under that threshold, you must get no errors. If you do, then you don’t meet the standards.
The only thing left then, is the cable that connects the input and the output devices. In order to ensure that the system behaves as intended, you are best to use a cable with the correct impedance. I will not get into what this means. If you are using AES/EBU over an XLR cable, then it should be a cable with a 110 Ω impedance. If you are sending S-PDIF over a coaxial cable, then it should be a 75Ω cable. If you do not use cables with the correct impedance, you will get some amount of reflection on the connection. However, the amount that you need to worry about this is proportional to the length of the connector. In other words, the longer the cable, the more you should worry about it.
Sampling Jitter
Sampling jitter will only happen:
- at the ADC
(which, for most people, means “at the studio when they did the recording – so there’s nothing I can do about it…” See the Footnote comment below…) - at the DAC
(which, for most people, means “at my output”) - or, in an poorly-implemented ASRC
(which, for most people, could be anywhere between those two – and probably happens multiple times through the chain)
The real question in the second and third of these cases is how good the device itself (the DAC or the ASRC) is at attenuating jitter. We can assume that jitter exists on the connections between devices – and inside devices. The real question is how well the device or components reduce the problem. For example, if you have a DAC that uses the incoming digital signal as the clock, and that external clock has jitter for some reason (we can assume that it does) , can the DAC reduce the timing errors? If it’s implemented well, then the answer is “yes”. It can smooth out the timing errors in the incoming sampling rate (using a PLL and/or an ASRC, for example) and create a new, clean clock.
In other words, if your source has jitter, but is within the standard for the transmission protocol, and your DAC is designed to attenuate jitter adequately, then the amount of jitter in the source is irrelevant (within reason).
However, if your DAC tracks the incoming sampling rate and uses it as the clock, and the source has jitter (but is within the standard) then the amount of jitter at the source’s output is not irrelevant.
So, unfortunately, there’s no simple answer that can tell you when you need to worry about jitter. It really depends on the specific abilities of your various devices and the components inside them.
Footnote: There is one notable exception to my statement that the ADC’s are the recording studio’s problem and not yours. This exception occurs when you have an analogue signal coming into a digital audio device. For example, if you have a turntable or a cassette deck going through a preamp or AVR with DSP. Another example is a loudspeaker with an analogue input, but DSP-based processing.
Can you hear jitter?
The simple answer to this these days is “probably not”.
The reason I say this is that, in modern equipment, jitter is very unlikely to be the weakest link in the chain. Heading this list of likely suspects (roughly in the order that I worry about them) are things like
- aliasing artefacts caused by low-quality sampling rate conversion in the signal flow (note that this has nothing to do with jitter)
- amateurish errors coming out the recording studio (like clipped signals, grossly excessive over-compression, and autotuners) (and don’t get me wrong – any of these things can be used intentionally and artistically… I’m talking about artefacts caused by unintentional errors.)
- playback room acoustics, loudspeaker configuration and listener position
- artefacts caused by the use of psychoacoustic CODEC’s used to squeeze too much information through too small a pipe (although bitrates are coming up in some cases…)
- Dynamic range compression used in the playback software or hardware, trying to make everything sound the same (loudness)
- low-quality loudspeakers or headphones (I’m thinking mostly about distortion and temporal response here
- noise – noise from the gear, background noise in your listening room… you name it.
So, if none of these cause you any concern whatsoever, then you can start worrying about jitter.
Jitter – Part 8.3 – Sampling Rate Conversion
#8 in a series of articles about wander and jitter
Although I am guessing, I don’t think that it is crazy to say that the majority of digital audio systems today employ some kind of sampling rate conversion somewhere in the signal flow.
A sampling rate converter is a physical device or a processing block in some software that takes an audio signal that has been sampled at one rate (say, 44.1 kHz) and converts it to an audio signal at another rate (say, 48 kHz).
There are many reasons why you might want to do this. For example, if you have a device that has equalisation (filtering), then if you change the sampling rate, you will have to new coefficients into the filters. If you have a LOT of filters, then it might take so much time to load them into the system that you’ll miss the first second or two of a song if it’s a different sampling rate than the previous song. So, instead of doing this, you keep your processing at one constant (or ‘fixed’) sampling rate, and convert the input to that rate. This might even be true in the case where the incoming sampling rate is the same as the internal sampling rate. For example, you might be “sample rate converting” from 48 kHz to 48 kHz – just to keep the design of the system clocking constant.
Looking very broadly, there are two options for sampling rate conversion.
Synchronous Sampling Rate Conversion
Let’s say that you have to convert from 48 kHz to 96 kHz – a multiplication of 2. In this simple case, you could take the incoming samples, and insert an new, extra one mid-way between each of them. The value of the new sample depends on how you are doing the math to calculate it. We will not discuss this here. The important thing about this concept is that the timing of the output is “locked” to the input. In this example, every second sample of the output happens at exactly the same time as every sample at the input. This can also be true if the ratio of the sampling rates are not “nicely” related like a 2:1 ratio. For example, if you have an input at 44.1 kHz and and output at 48 kHz, you could take the incoming 44.1 kHz signal, insert 47999 “virtual” samples between each of the original samples (making the new sampling rate 2116800000 Hz) and then pull an output sample from that stream every 444100 samples.
In other words:
(44100 * 48000) / 44100 = 48000
Of course, this is not a smart way to do this (because it will be a huge waste of processing power and memory – and imagine how big the numbers would be if you’re converting 176.4 kHz to 192 kHz… bigger!), but it would work, as long as the “virtual” samples you create at the very high “virtual” sampling rate have the correct values.
This type of sampling rate conversion, where the output is numerically “locked” to the input in time (meaning that, at some regular interval of time, the input and the output samples will happen simultaneously – or at least with a constant delay) is called synchronous sampling rate conversion. It’s called that because the input and the output are synchronised with each other… A bit like gears meshing together.
Asynchronous Sampling Rate Conversion
There is another way to do this, where we do not lock the output clock to the input clock. Let’s say that you want to build a device that has a constant sampling rate at its output, but you don’t really know what the sampling rate of the input is. In this case you will use an asynchronous sampling rate converter – so-called because there is no fixed lock between the input and output clocks.
In this case, the incoming signal is analysed and its sampling rate is measured. The way this is done is a little similar to the method shown above. You take the clock running at the rate of the output’s signal and multiply that by some value (say 512, for example) to create an internal “virtual” clock running at a higher sampling rate. You then “grab” the value of an incoming sample and apply its value to the “virtual” sample that is closest in time. This allows the incoming samples to drift in time relative to the output samples.
In both cases, there is the open question of how you generate the signal at the higher internal sampling rate. This can be done using a kind of low pass filter that is effectively similar to the reconstruction filter in a DAC. I will not talk about this any more than that – other than to say that the response characteristics of that filter are VERY important… So, if you’re planning on building your own sampling rate converter, read a lot more stuff on the subject than what I’ve written here – because what I’ve written here is most certainly not enough information.
There’s one strange effect that pops up here. Since, in an ASRC (Asynchronous Sampling Rate Converter) the incoming signal is sampled at discrete times that are numerically related to the output sampling rate, then any potential jitter in the system is also quantised in time. So, for example, if your output sampling rate is 48000 samples per second, and you’re creating the internal sampling rate by multiplying that by 512, then any jitter in the ASRC cannot have a value less than 1/(48000*512) second = 4.069*10^-8 or 40.69 nanoseconds. In other words, in such a system, the error caused by jitter will be 0, ±40.69 nanoseconds, ±81.38 nanoseconds, and so on. It can’t be something in between… (assuming that the output clock is perfect. If it’s drifting due to jitter, then those values will also drift…)
The good news is that, if the clock that is used for ASRC’s output sampling rate is very accurate and stable, and if the filtering that is applied to the incoming signal is well-done, then an ASRC can behave very, very well – and there are lots of examples of this. (Sadly, there are many more examples where an ASRC is implemented poorly. This is why many people think that sampling rate converters are bad – because most sampling rate converters are bad.) in fact, a correctly-made sampling rate converter can be used to reduce jitter in a system (so you would even want to use it in cases where the incoming sampling rate and outgoing sampling rates are the same). This is why some DAC’s include an ASRC at the input – to reduce jitter originating at the signal source.
Wrapping up Part 8: The take-home messages for these three parts in Section 8 are:
- Sampling Jitter results in some kind of distortion of the signal that can be related to the signal itself
- Sampling Jitter can occur in the ADC, the DAC, or an ASRC
- If implemented correctly, an ASRC can be used to attenuate jitter in a system
- Once introduced to the signal, jitter cannot be attenuated. So, if you have a recording that was made using an ADC with a lot of jitter, the artefacts caused by that jitter is in the recorded signal forever. If you have a DAC that has absolutely no jitter whatsoever (this is not possible) then this will not eliminate the jitter that is already in the signal. Of course, it won’t make the situation worse… but it won’t make it better.
Addendum. If you want to dig further into the world of Sampling Jitter and the advantages of using ASRC’s to attenuate jitter, I highly recommend the following as a good starting point:
- Julian Dunn’s paper called “Jitter Theory” – Technical Note TN-23 from Audio Precision. This is a chapter in his book called “Measurement Techniques for Digital Audio”, published by Audio Precision. See this link for more info.
- Clock Jitter, D/A Converters, and Sample-Rate Conversion
By Robert W. Adams, Published in The Audio Critic, Issue No. 21 - The Effects of Sampling Clock Jitter on Nyquist Sampling Analog-to-Digital Converters and on Oversampling Delta Sigma ADCs, Steven Harris. AES Preprint #2844 (87th International Convention of the AES, October 1989)
- Jitter Analysis of Asynchronous Sample-rate Conversion, Robert Adams. AES Preprint #3712 (95th International Convention of the AES, October 1993)